The Down-Low on Wind Shear
There are some things your CFI may not have taught you from the TAF.
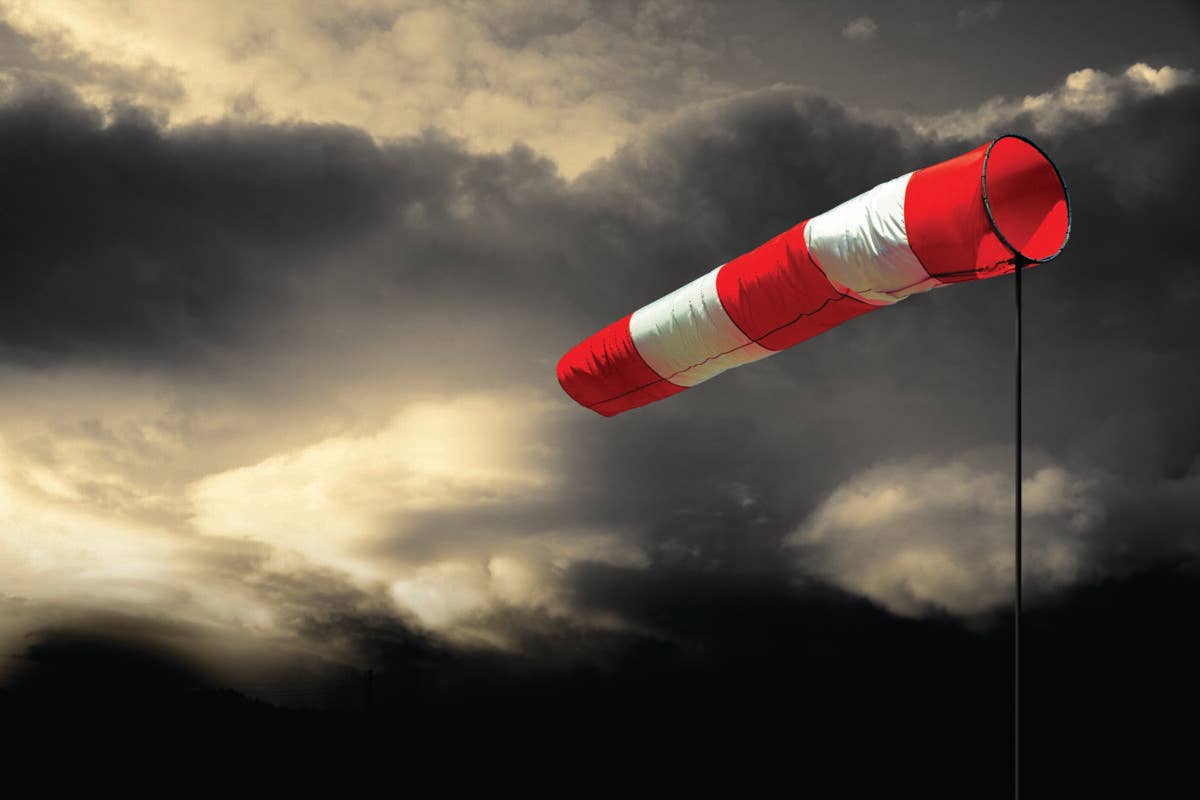
TAFs are one way to identify the likelihood of nonconvective low-level wind shear for your departure or destination airport. [Adobe Stock]
There’s no doubt that terminal aerodrome forecasts, simply known as TAFs, are perhaps the most detailed aviation forecasts available to a pilot. Within them lays a piece of forecasting critical to understand, and that’s for low-level wind shear.
If you call Flight Service for a standard briefing or get an automated briefing through one of the many heavy-weight apps, you can bet the farm that any TAFs along your proposed route and at your departure and destination airports will be a part of this briefing. There are, however, some finer details about the forecast found in TAFs that instructors fail to pass along to their primary students possibly owing to their own lack of knowledge. The top one on the list includes a forecast for nonconvective low-level wind shear (LLWS).
What Your CFI Didn’t Teach
A forecast for nonconvective LLWS is probably the most misunderstood aviation forecast among pilots and instructors. In a TAF, this forecast appears in coded form with a WS code such as WS020/15055KT. Such a forecast can also appear in a graphical AIRMET (G-AIRMET) issued by forecasters at the Aviation Weather Center (AWC). In a preflight briefing, pilots hear the term “wind shear” and immediately equate this with thunderstorms and severe turbulence. It’s a common misconception, but nonconvective LLWS as it appears in a TAF is not a forecast for turbulence at all. In fact, in most cases when this is forecast, the air is glassy smooth.
With a large-scale weather system, this form of windshear is quite common on either side of a warm front for a developing or occluded area of low pressure. But it’s also quite prevalent in the overnight hours during fair weather conditions coupled with a clear sky and calm wind at or near the surface.
If you're not already a subscriber, what are you waiting for? Subscribe today to get the issue as soon as it is released in either Print or Digital formats.
Subscribe NowEven though wind seems to be the common denominator in this description, atmospheric stability is the catalyst behind most nonconvective LLWS occurrences. We know that wind naturally tends to increase in speed with increasing height, but it normally does so gradually, especially in the latter part of the afternoon when the boundary layer near the surface is well mixed. But what if the winds are light or nearly calm at the surface and increase to 55 knots just 1,500 or 2,000 feet above the ground? That’s an example of vertical speed shear and is known as nonconvective LLWS.
When the winds are expected to increase rapidly with height within 2,000 feet of the airport’s surface, it is common that a forecast for nonconvective LLWS will be issued in a TAF for that airport. Such a forecast tells the pilot about the potential for the wind speed to increase rapidly with increasing height above the ground within a shallow layer called the wind shear layer. That is, faster air at the top of the wind shear layer is moving over slower air near the base of that layer. There also may be an accompanying shift in wind direction with altitude in this layer, but it is the vertical speed shear that is the primary trigger for the forecast.
- READ MORE: The Points Forecast Sheds New Light on TAFs
Not Like a Thunderstorm
Keep in mind that this is not the same horizontal and vertical wind shear that may be experienced in the vicinity of deep, moist convection or thunderstorms, hence the name nonconvective LLWS. In fact, forecasts for convective and nonconvective LLWS have very distinct differences. In a TAF, convective LLWS will typically contain a reference to showers or thunderstorms (e.g., SHRA, TS, VCTS) and will contain CB—which stands for cumulonimbus—in the cloud group. Also, the surface winds are typically forecast to be strong and gusty. While convective LLWS can occur at any time of the day or night, most occur in the afternoon and early evening when convection is the most prevalent, especially west of the Continental Divide. Here are three examples of forecasts for convective LLWS that can be found in a TAF:
- FM132200 33010G20KT P6SM VCTS SCT015 BKN040CB
- FM131600 22013G35KT 3SM TSRA BR BKN035CB
- FM140000 VRB20G55KT 1/2SM +TSRA FG BKN015CB
Nonconvective LLWS can occur in the cool or warm sector of an area of low pressure, but it can frequently take place in the presence of a strong nocturnal surface-based temperature inversion. Frontal nonconvective LLWS can happen any time of the day or night and normally possesses the characteristics of light wind sat the surface and cloudy skies but can be strong and gusty when the weather system is associated with an intense occluded area of low pressure. Here are three examples of TAFs for nonconvective LLWS associated with a frontal system:
- FM111600 13010KT 5SM -RA OVC015 WS020/27055KT
- FM120100 VRB03KT 4SM BR OVC008 WS015/25045KT
- FM120900 19018G30KT 3SM +SHRA BR OVC005WS020/17075KT
However, nocturnal nonconvective LLWS occurs in the overnight or early morning hours often with a light wind and clear sky. This is a manifestation of radiation cooling and likely occurs in the region under an area of high pressure. Here are three examples of the nocturnal version of nonconvective LLWS in a TAF:
- FM221100 19004KT P6SM SKC WS015/17040KT
- FM230800 VRB03KT P6SM SCT010 WS010/22035KT
- FM230400 00000KT P6SM SKC WS020/23055KT
Where to Find It
In both cases of nonconvective LLWS, the LLWS code—WS—will be included in the TAF immediately after the cloud group. Let’s take a closer look at this misunderstood forecast group. Assume the following from a TAF:
- FM252300 15014G25KT P6SM SKC WS020/16055KT
The first element to the immediate right of the WS code is a height above the airport—in this case 020 or 2,000 feet. This represents the maximum depth of the wind shear layer.
This altitude is “typically” one of four values—005 for 500 feet agl, 010 for 1,000 feet agl, 015 for 1,500 feet agl, or 020 for 2,000 feet agl. Even if the wind shear layer extends higher, the maximum height that is forecast is 2,000 feet.
Following the forward slash, the next group contains the true wind direction followed by the wind speed in knots at the indicated height, or 160 degrees at 55 knots in this example. This implies indirectly that the wind is rapidly increasing from the surface through the indicated height, although this says nothing about the wind direction throughout this shear layer. Effectively this forecast translates into “the wind at 2,000 feet agl is 160 degrees at 55 knots.” But it does not imply there will be turbulence at 2,000 feet agl or below. In most cases, you’ll find smooth conditions in this wind shear layer, especially for the nocturnal instance of nonconvective LLWS.
A Question Of Stability
The catalyst for the development of nonconvective LLWS is atmospheric stability. We also know that temperature normally decreases with increasing altitude. This is generically referred to as a lapse rate—simply a change of temperature over a change of increasing altitude. Anytime the temperature decreases with increasing altitude, it’s referred to as a positive lapse rate. If the temperature increases with altitude, that’s referred to as a negative lapse rate or more commonly called a temperature inversion. The larger the lapse rate, the greater the atmospheric instability. An unstable environment (large lapse rate) promotes vertical mixing and provides for a more turbulent air flow potential. On the other hand, a stable atmosphere (small or negative lapse rate) inhibits vertical mixing and provides for a laminar and nonturbulent flow.
One might suspect that vertical speed shear (faster air flowing over slower air) could cause the air to overturn and produce turbulent eddies within this windshear layer. Think about a tumbleweed rolling across the ground somewhere in the southern Plains. However, just about all nonconvective LLWS occurrences feature a surface-based, low-level temperature inversion. Any kind of overturning or vertical mixing introduces the potential for turbulence, however, an extremely stable layer such as this tends to dampen or resist vertical mixing.
So why does the air accelerate rapidly with height? The extreme stability courtesy of the inversion eliminates upward and downward motion or vertical mixing (neutral buoyancy). This promotes a laminar flow, and the effects of surface friction are no longer “felt” at heights a few hundred feet above the surface. This allows the flow of air just above the treetops to accelerate uninhibited and insulated from surface friction below through the depth of the wind shear layer.
You can think of this as a faster flowing river of air (called a low-level jet) located just above the surface. The stronger and deeper the inversion, the less likely there will be any kind of turbulence.
Why It’s Forecast
So, if nonconvective LLWS isn’t a forecast for turbulence, why is it forecast at all? When the sky is clear and surface winds are light, the nocturnal version of this phenomenon is just as common as low-level thermal turbulence in the afternoon during the warm season. Unless you were fixated on your groundspeed approaching an airport late at night or in the early morning hours, you probably flew right through it without even noticing that it existed. In most cases, nocturnal nonconvective LLWS isn’t typically forecast.
Nevertheless, there are several situations where you should pay close attention. First, if you are departing out of an airport with a high density altitude, nonconvective LLWS can make for a difficult climb if the low-level jet is off your tail. It’s not uncommon for the winds to be light or calm at the surface although they may be 30 knots or more just above the tree tops. With light or calm winds at the surface, you may not realize that during the initial climb to pattern altitude, the prevailing wind is at your back. If you pilot a balloon, you should pay close attention to these forecasts. A hot air balloon is not a rigid body and can deform easily in the presence of these conditions.
The most important one to watch out for is when nonconvective LLWS of 50 knots or greater is coupled with the potential for moderate to heavy rain showers (SHRA or +SHRA) or even thunderstorms (TSRA or +TSRA). Yes, both convective and nonconvective LLWS can be forecast at the same time.
As the moderate to heavy rain falls through the low-level jet, some of the momentum of the jet gets transferred or directed toward the surface of the earth. This is like taking a fire hose and deflecting it downward toward the ground. The downward momentum of that low-level jet creates the potential for wet microbursts or downbursts.
In this case, the magnitude of the nonconvective LLWS event and convective outflow can make for a real interesting approach to land. If nonconvective LLWS is forecast and the atmosphere in the windshear layer is not as stable, then you might experience a rough ride.
What if There Isn’t a TAF?
TAFs are one way to identify the likelihood of nonconvective LLWS for your departure or destination airport. However, not all airports are served by a TAF. Meteorologists at the AWC also issue a forecast for nonconvective LLWS. These are issued for areas that cover at least 3,000 square miles, or roughly two-thirds the size of Connecticut. You’ll see this as part of the routine issuance of a graphical AIRMET (G-AIRMET). While the forecast depth of the wind shear layer may vary in a TAF, the wind shear layer in a G-AIRMET is fixed at a depth of 2,000 feet agl.
Wind shear in a preflight briefing is often equated with thunderstorms and severe turbulence. Understandably, it’s common for many pilots to become anxious when they see such a forecast. But some forms of wind shear might not always be dangerous or even problematic to light aircraft. When nonconvective low-level wind shear (LLWS) exists, for example, the air maybe glassy smooth. This is especially true when you see a forecast for nonconvective LLWS in the overnight hours that’s not associated with a large-scale weather system.
It’s not a forecast that should instill fear in a pilot like it often does. In most cases, it will be a nonevent that you may not even notice if you missed it in your preflight briefing. Even so, always keep a close watch on pilot weather reports for turbulence.
This column first appeared in the July 2023/Issue 933 print edition of FLYING.
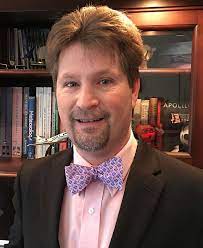

Subscribe to Our Newsletter
Get the latest FLYING stories delivered directly to your inbox