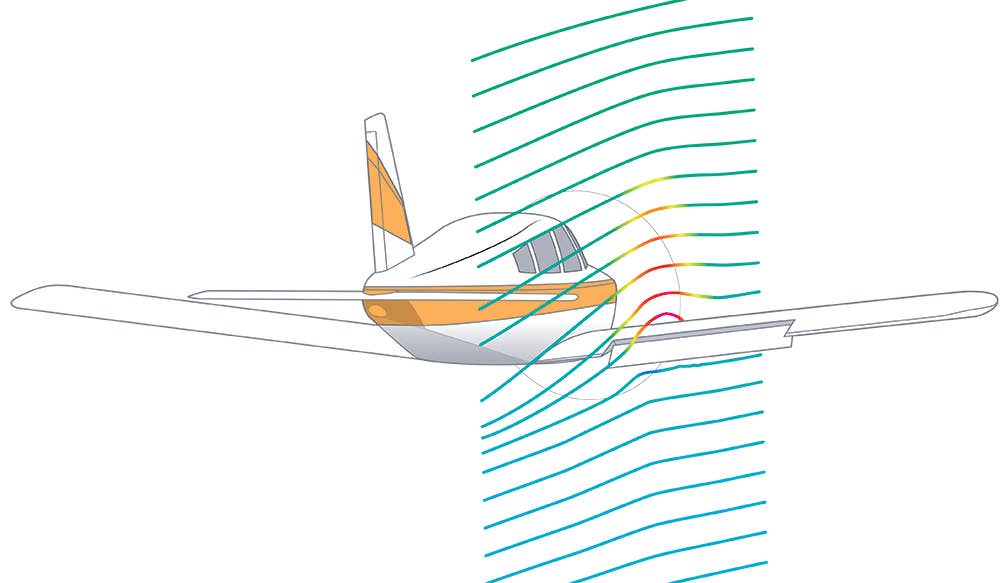
It is useful to practice a few balked landings, at a safe altitude, to see firsthand how your airplane behaves and how you yourself react. Tim Barker
Writing last month's Aftermath column about a fatal accident that resulted from the pilot's mishandling of a balked landing, I reflected that I had never assessed the behavior of my own airplane in that maneuver. As I have said before, my idea of flight testing is to take trips and wait for something strange to happen, and as luck would have it, I have never yet had a balked landing in Melmoth 2.
In the Aftermath accident, the pilot selected full flaps, allowed speed to decay to the vicinity of the stall and then, thinking she was still too high to land, decided to go around. She retracted the flaps without first gaining speed. The minimum flying speed went up along with the flaps, and the airplane, a Cirrus, stalled and spun.
This seems like an elementary error, but changing configuration at low speed is not always an easy thing to do. Early Cessna 150s and 172s, for instance, were notoriously unable to gain speed with full flap; you had to bleed the flap up very carefully while you waited for the diminishing drag to allow the airplane to very gradually accelerate. If you had to turn or climb to avoid an obstacle, you were in a bad fix. This characteristic was not confined to low-powered personal airplanes; the Boeing 727, with its fantastically complicated and powerful triple-slotted flap, had it too. Both the Cessnas and the Boeing had 40-degree flap positions that many operators eventually disabled because of the drag they entailed.
The first airplane I built, Melmoth (1973-1982), had a double-slotted Fowler flap that deflected 45 degrees. It produced a tremendous amount of drag. One of the tricks with which I would amuse or appall passengers was to stay at pattern altitude on final approach until the runway disappeared from view under the nose.
I would then chop the power and land on the numbers. On one memorable occasion, with Mike Melvill riding in the right seat, I began the flare a little too abruptly and the nose did not come up. Thus, in accordance with my philosophy of flight testing by random events, I learned that with a forward CG and full flap, the stabilator could stall.
Flaps 40 on those early Cessnas also produced a powerful nose-down pitch. You would trim to approach speed with full flap, and only then discover that the Creator had omitted to provide you with a third hand with which to retrim while the left hand pushed like mad on the yoke and the right milked the flap lever.
I expected my second design, Melmoth 2 (2002-), to present similar problems. It has large Fowler flaps — but only single-slotted, and limited to 30-degree deflection — retractable landing gear and an airbrake under the belly, near the leading edge of the wing. I decided that the go-around procedure should begin with a power increase, followed by gear retraction, then the airbrake (which is always used for landing) and finally the flaps.
At altitude, I configured for landing and set up a 500 fpm descent at an approach speed of 73 knots. This required about 18 inches of manifold pressure. I then pretended that an elk had strolled onto the imaginary runway in front of me. I arrested the descent while bringing the power up to 26 inches (the maximum allowable is 41) and started cleaning up.
It was quite a complex routine. There was no pitch trim change with gear retraction but a marked, and sudden, pitch down with airbrake retraction. There was an equally marked, but more gradual, pitch up between flaps 30 and flaps 10, and very little change the rest of the way. With me alone in the airplane it’s at its most forward CG, and has just enough trim authority to maintain a 1.3 Vs approach speed with flaps and airbrake down. Trim is manual, so when the airbrake came up, I had the same problem as the Cessna 150 pilot: not enough hands. As I cleaned up, therefore, I was making all the adjustments with the sidestick. It wasn’t smooth.
I would have lived, but might have given the elk a scare.
Go-around behavior varies among types, largely because of the trim changes associated with flaps. Flap deflection, because it increases the camber of the wing, eventually results in a more nose-down fuselage attitude if you hold speed constant. But it is usually necessary to retrim, and the transitional trim change while the flap is moving may be nose up or nose down.
The trim change is due to two factors. The first, which is common to all flapped wings, is an increase in the nose-down force generated by the wing itself. All cambered wings (unless they have reflexed trailing edges) produce a nose-down force that is counteracted by the stabilizing surface, be it a horizontal tail or a canard. A deflected flap, which increases camber, greatly augments that force.
In addition, the moving flap — generally, the wings of canard airplanes don’t have flaps, so we’re just talking about tail surfaces now — changes the angle of airflow at the tail. That change is invariably nose up because the downward angle of the wing’s wake pushes the horizontal tail downward.
So, the reaction to flap deflection is the sum of the nose-down force of the wing and the nose-up force due to the downwash at the tail. Each varies at its own rate with flap deflection, and in a given configuration either may predominate.
The wing’s wake can be thought of as a capelike sheet emanating from the trailing edge. The downwash angle is greatest along that sheet; above and below it, the downward displacement of the wake lessens and, at a distance of a half span or so, eventually disappears.
In the accompanying illustration, which is a computer analysis of the downwash a few feet out along the wing with the flap deflected, you can see that the downwash angle at the tail is greatest at a point somewhat lower than the wing. That is why airplanes, for example all high-wing Cessnas, whose horizontal stabilizers are below their wings, usually require nose-down trim with flap deflection, whereas low-wing airplanes, whose horizontal tails are farther from the center of the wake and encounter a lesser downwash angle, are more likely to require nose-up trim. The difference is magnified by the fact that the fuselage, which disturbs the downwash in its own wake, is less disruptive in high-wing than in low-wing airplanes. Power effects further complicate the picture, producing particularly marked pitch up in a single-engine high-wing, low-tail airplane.
This is a simplification. The relations among wings, flaps, fuselages and tails vary in many ways, and a particular airplane may violate the general rule. That is why it is useful to practice a few balked landings, at a safe altitude, to see firsthand how your airplane behaves and how you yourself react. If you doubt your own test-piloting abilities, do it during your next flight review, under the supervision of an instructor. The behavior of your airplane at low speed, with sudden application of power and changes in flap setting, might surprise you.


Subscribe to Our Newsletter
Get the latest FLYING stories delivered directly to your inbox